Wire myography: the ultimate guide [protocol included]
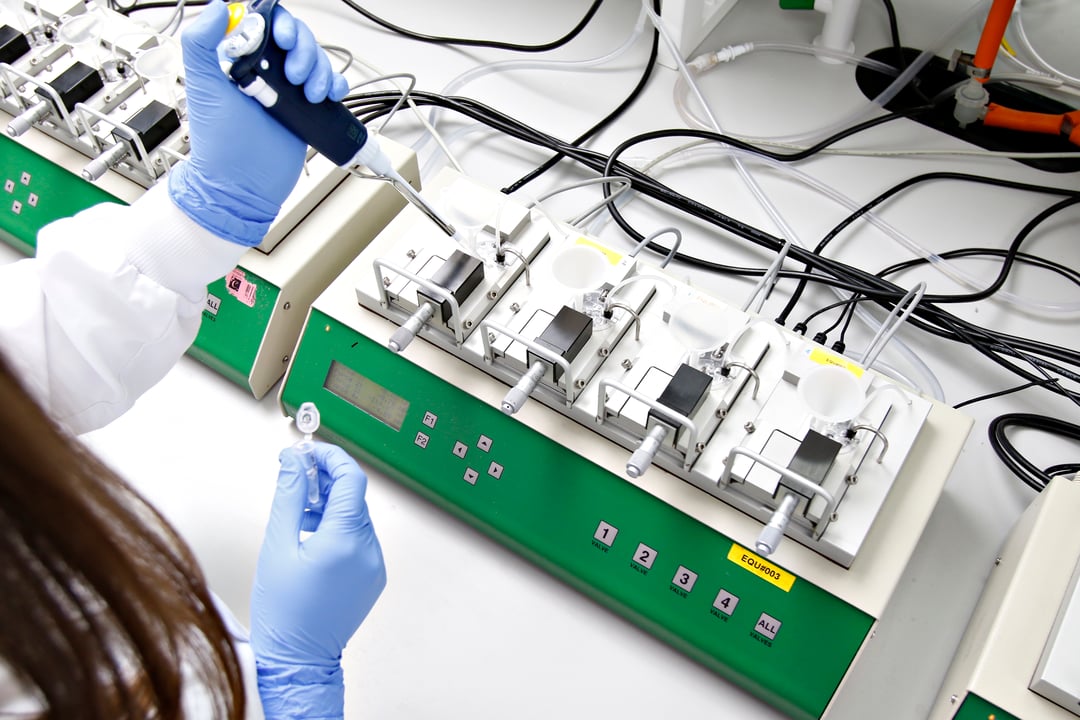
In the late 1970s, Dr Mike Mulvany and Dr William Halpern developed various new techniques to better investigate small blood vessel function, including the wire myograph system. This new laboratory apparatus was designed for investigations into the physiology and pharmacology of blood vessels that were too small to mount in a traditional organ bath, meaning researchers could readily investigate intact isolated arteries smaller than 1-2 mm in diameter in an in vitro system for the first time.1,2
Today, there are several companies that manufacture these systems, and one specialist contract research organization (CRO) that offers wire myography as a service. This article summarises this unique apparatus, including a protocol for using resistance arteries in the wire myograph system.
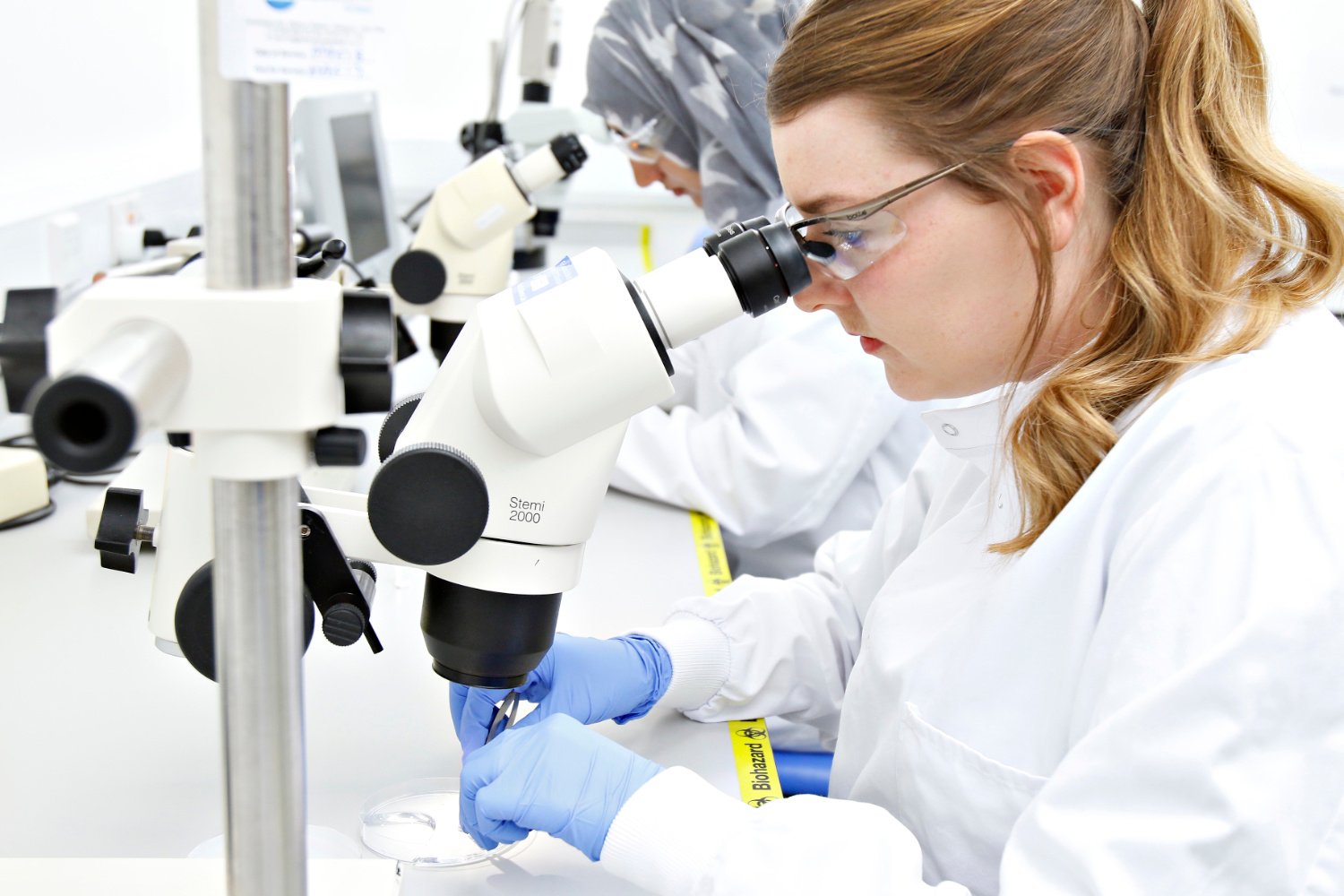
REPROCELL’s contract research services use human living tissues to better predict drug safety and efficacy.
What is a wire myograph?
A wire myograph is a type of laboratory apparatus used to measure changes in contractile force of luminal tissue segments under isometric conditions. Together with perfusion myography, it is considered the gold standard for measuring vasoactivity in small blood vessels between 100 μm to 2mm in diameter. It can also be used to estimate bronchoconstriction and bronchodilation in airways of a similar size.1,2 Multi-myograph systems are most commonly used as they can measure the contractility of several tissues simultaneously, with each isolated tissue set up in a separate tissue bath.2
History of the wire myograph system
Detailed diagrams of the first-ever wire myograph were revealed in "Contractile Properties of Small Arterial Resistance Vessels in [...] Rats," a seminal paper authored by Mulvany and Halpern in 1976.3 The structure of this original system was based on a technique pioneered by Bevan and Osher to measure arterial contractility ex vivo.3,4
Prior to the mid-70's, there was no laboratory apparatus that could readily measure the contractility of arteries smaller than 1-2 mm in diameter. Most vascular studies were performed in organ bath systems using large diameter aortic and conduit artery tissues, which have a limited effect on blood pressure.3 The invention of the wire myograph meant that researchers could investigate the effect of drugs on small arteries and veins including resistance arteries: small blood vessels that, because they are so numerous and are capable of significant vasoconstriction and dilatation, control up to 90% of total peripheral resistance (TPR) and therefore have a significant impact on the minute-by-minute regulation of systemic blood pressure.3,5
What tissues can be used in the wire myograph system?
Wire myographs can be used to measure drug effects on a range of isolated tissue types independent from homeostatic mechanisms, such as blood flow.6 These (healthy or diseased) samples are used as soon as possible after dissection to maintain their functionality and reflect an accurate tissue response:2,3
- Human tertiary or lower airways
- Canine mesenteric arteries
- Human pulmonary arteries
- Rat aorta
- Rat mesenteric arteries
- Human subcutaneous resistance arteries
- Human small coronary arteries
Structure of the wire myograph system
The basic structure of the wire myograph has remained unchanged since 1976, although the instrumentation itself is now more robust and allows digital capture of force measurements. Each chamber is approximately 8 mL in volume (typically filled with 5 mL of fluid) and contains two opposing steel jaws. The first jaw is attached to a movable micrometer which allows researchers to precisely stretch the tissue to a physiologically relevant pre-load. The second jaw is linked to an isometric force transducer that sensitively measures the ability of the tissue to contract against a given transmural pressure.3
The transducers are incredibly sensitive and are capable of measuring micro-Newton changes in force, detecting even the smallest changes in contraction or relaxation of the isolated blood vessel or bronchi. To provide a meaningful comparison of how small these forces are, one apple exerts a force of about two Newtons at the earth's surface (one Newton being the force required to accelerate 1 kg by 1 meter per second).
The isolated tissue segments are carefully attached to the jaws via two wires threaded through their lumen (figure 1). This procedure requires extensive practice, with skilled operators able to set up arteries while avoiding damage to the sensitive endothelial layer. Most myographs also allow the use of larger tissues (greater than 1-2 mm diameter) by replacing the jaws with conventional stainless steel hooks, effectively converting the wire myograph to an organ bath.
Figure 1: Diagram showing the structure of the myograph jaws and how luminal tissues are mounted between the wires.
Examples of wire myograph use in the literature
In drug discovery, wire myographs are most commonly used in late-stage nonclinical studies to estimate the effect of drugs on blood pressure and/or flow. For example, they are used in cardiovascular safety studies to detect potential clinical adverse effects on the cardiovascular system and can be conducted in compliance with GLP guidelines as part of supplementary tests in line with ICH S7A safety pharmacology studies. Less frequently, as fewer drugs for hypertension are in development than in the past, wire myography is used to measure drug efficacy, where the therapeutic aim is to increase or decrease vascular contractility. We have listed papers where wire myography has been used by Pharma to predict drug responses below:
- Skinner M et al. The contribution of VEGF signaling to fostamatinib-induced blood pressure elevation. British Journal of Pharmacology 171 pp 2308-2320 (2014).
- Wong MD et al. Recombinant Human Elastase Treatment of Cephalic Veins. Cardiovascular Pharmacology 5:2 (2016).
Wire myography protocol: Measuring the effect of drugs on blood pressure using small resistance vessels
Small changes in the contractility of the resistance vasculature can significantly alter blood pressure and flow. These vessels are, therefore, often used in wire myograph experiments to estimate drug effects on blood pressure.2,5 Throughout the entire culture period, the myograph chamber is filled with a 37°C nutrient solution (typically a physiological saline solution (PSS) such as Krebs-Henseleit solution) that is aerated with oxygen (95%) and carbon dioxide (5%).1,2
The first step of this process involves dissecting the arteries from the surrounding tissue, which is often rat mesenteric tissue. Once isolated the resistance vessels are mounted in the myograph, stretched to a physiologically relevant pre-load, and left to equilibrate for approximately 40 minutes.2 Tissue viability is then tested using a series of depolarisations with high potassium physiological salt solution (KPSS)2 and endothelium integrity is checked with acetylcholine (ACh).
Once tissue viability is confirmed, drugs can be added to the bath and the resulting response (contraction/relaxation) displayed on a computer monitor.1 Raw data from the myograph trace can then be used to plot a cumulative concentration-response curve (CCRC) and calculate the half-maximal effective concentration (EC50) of the compound.2 If you are looking for a protocol describing this process in more detail we have included one below.
Stage 1: Isolating resistance arteries from tissue
- Place the entire tissue in a petri dish containing ice-cold physiological saline solution (PSS)
- Isolate the arteries from the tissue under a dissecting microscope using surgical scissors and forceps.
- Divide the isolated vessels into four 2mm segments (if using one four-channel wire myograph system) and remove any excess adipose and/or connective tissue before mounting.
Stage 2: Mounting tissue segments in the wire myograph
- Prepare each myograph chamber by washing them three times with dH2O and three times with ice-cold PSS.
- Add 5 mL of PSS to each wire myograph chamber.
- Under the microscope, attach the first wire, to the left myograph head. Then gently slide the vessel onto the wire through the lumen and then attach the second end of the wire to the other side of the jaw.
Note: Your myograph manual should contain diagrams explaining the process for your specific system in more detail. - Using forceps, slide the second wire into the vessel lumen alongside the first wire. This should be performed as a single motion to avoid trauma to the vessel.
- Gently close the myograph jaws.
- Put the myograph chambers back on the main unit, switch on the gas and heating and allow it to reach 37°C, over at least a 30 minute period.
- Normalize the arteries, e.g. by using the DMT normalization module on Chart software. This sets the arteries to the optimal tension for their size.
- Wash each artery by emptying each bath and adding 5 mL of PSS. Repeat at least a further two times then allow tension measurements to stabilize.
- Once the tension measurements have stabilized, set the tension measured on each channel to zero.
Stage 3: Testing tissue viability
- Following the rest period, add 5mL of KPSS to each bath.
- Once the contraction response has plateaued, wash the tissue with PSS and allow it to relax to baseline. Repeat this process three times.
- Add norepinephrine (or another suitable vasoconstrictor) to the bath. When the contraction peaks, add acetylcholine (ACh) or another suitable endothelium-dependent vasodilator to test the vasodilator response of the tissues and assess that the endothelium is intact and functional.
Constructing a cumulative concentration-response curve (CCRC)
A cumulative concentration response curve (CCRC) is an experimental protocol used to observe how the concentration of drug present at the tissue relates to the drug effect at each concentration, when drugs are added cumulatively, without washes between drug additions. It allows the potency and magnitude of drug responses to be readily observed and allows the calculation of useful parameters such as the EC50, the concentration of drug that elicits 50% of the maximum response.2
To construct a CCRC, you must reach a maximum tissue response to the drug by gradually saturating the available target receptors. This is achieved by cumulatively increasing the concentration of a drug in the myograph bath, with each subsequent concentration being added only after the response to the preceding concentration has plateaued (figure 2).
This is in contrast to a non-cumulative concentration response curve, where the tissue is washed between drug additions and the artery, vein or airway returns to a baseline force. Non-cumulative concentration response curves are in practice often difficult to execute, because frequent washing of the tissue can disrupt the baseline force measurements and make data interpretation more difficult; therefore, cumulative concentration curves are most often preferred.
Figure 2: Example of a continuous data trace obtained from a myograph experiment used to construct a CCRC.
Vasodilation versus vasoconstriction CCRCs
CCRCs to explore the vasoconstrictor or bronchoconstrictor effects of a drug are most often conducted from the baseline force of the tissue. In order to observe vasodilatation/vasodilation or bronchorelaxation, however, it is typical to "pre-constrict" the tissue, to increase the level of force and provide a window against which relaxation can be observed. This is because the resting tone in most tissues, in the absence of any exogenous contractile agent, is relatively low and the addition of a vasodilator/bronchodilator may not be clearly observed. Airways do tend to have a higher endogenous tone and it may not always be suitable to pre-constrict the tissues; therefore, the protocol to be used requires careful consideration.
Before constructing a vasodilation or bronchodilation CCRC, the tissue should therefore be contracted to approximately 80% of its maximum response using a suitable agonist e.g., norepinephrine which produces a stable constriction against which any relaxant effects can be observed. In the typical experimental set-up on a wire myograph, note that "vasodilators" or "bronchodilators" don't actually dilate the tissue, as it is an isometric experiment where the stretch of the tissue; instead vasodilators or bronchodilators relax the tissue, reducing the observed force.
The agonist used to induce relaxation should be added again once the tissue response plateaus. After a maximum response is achieved (there are no further responses observed to the drug additions), the tissue can be washed with PSS and exposed to additional compounds. It is important to plan the order that the compounds are added to the bath, adding drugs with irreversible effects last, as these cannot be washed out.2
If creating a vasoconstrictor CCRC, you must leave time between drug additions to allow the vessel to contract fully. When this contraction response has peaked the next concentration can be added and the process is repeated until a maximum contraction is reached.
Conclusion
The wire myograph is a highly sensitive laboratory apparatus that can detect small changes in force, as a way to observe the contractile and relaxant state of isolated fresh tissues. It can be used to measure the ex vivo contractility of arteries, veins or airways less than 2 mm in diameter, including small resistance arteries with diameters in the range of 200 to 500 microns.
If you wish to outsource wire myography or organ bath experiments for drug development, our researchers have since 2002 been providing specialist ex vivo research using a wide range of arteries and airways, including GLP-compliant safety pharmacology studies. We are highly skilled in fresh tissue dissection and can access ethically-donated human tissues through our specialized tissue network. You can find out more about our wire myograph studies here, or contact our team if you have any questions.
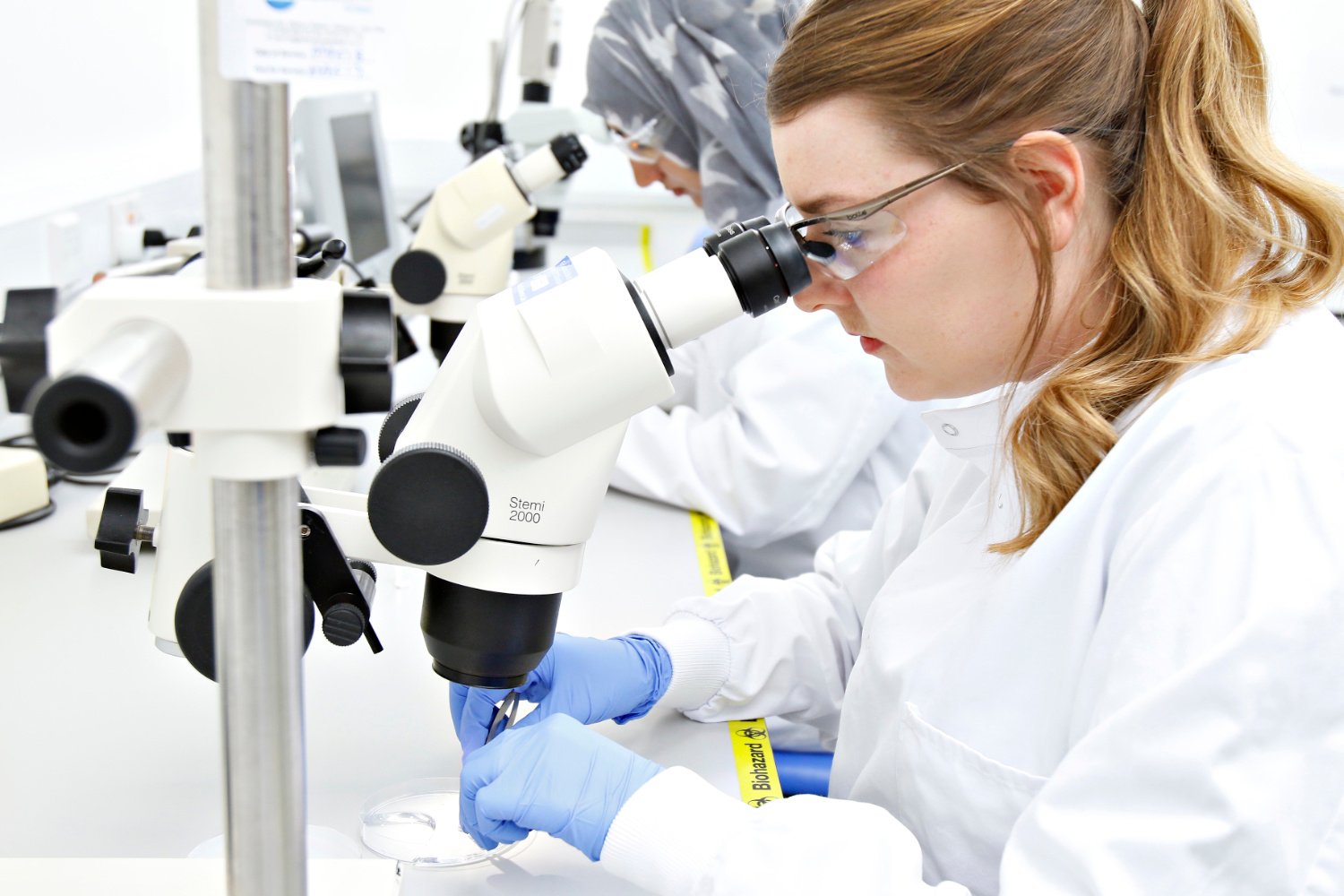
REPROCELL’s contract research services use human living tissues to better predict drug safety and efficacy.
References
- Olson KR. Design and Physiology of Arteries and Veins | Physiology of Resistance Vessels. Encyclopedia of Fish Physiology pp 1111-1118 (2011).
- Spiers A et al. A Guide to Wire Myography. Methods in Molecular Medicine 108 pp 91-104 (2005).
- Mulvany MJ et al. Contractile Properties of Small Arterial Resistance Vessels in Spontaneously Hypertensive and Normotensive Rats. American Heart Association Journals 41 pp 19-26 (1977).
- Bevan JA et al. A Direct Method for Recording Tension Changes in the Wall of Small Blood Vessels in vitro. Agents and Actions 2:5 pp257-260 (1972).
- Lee R et al. Vascular structural and functional changes: their association with causality in hypertension: models, remodeling and relevance. Hypertension Research (2017).
- Angus JA et al. Techniques to study the pharmacodynamics of isolated large and small blood vessels. J Pharmacol Toxicol Methods 44:2 pp 395-407 (2000).
- Skinner M et al. The contribution of VEGF signalling to fostamatinib-induced blood pressure elevation. British Journal of Pharmacology 171 pp 2308-2320 (2014).
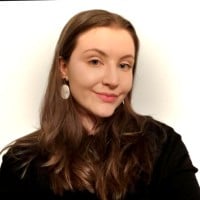
Author
Zara Puckrin, BSc
Zara is a GCU graduate who loves minimalism, marketing, and molecular biology. You can contact her on LinkedIn.
Subscribe to receive updates from REPROCELL
Stem cell and drug discovery scientists around the world are using REPROCELL’s services and products in their preclinical and clinical research.