Before a new drug is tested in humans pharmacologists must ensure that any potential cardiovascular harm is reduced or eliminated.1,2 Any drug-induced changes to blood pressure, heart rate, or electrical activity represent a significant safety concern, particularly in vulnerable patient populations.
Cardiovascular safety pharmacology is an area of drug development that aims to:
- Detect adverse drug effects on the heart and blood vessels before clinical trial1
- Determine the cardiovascular safety margin of new drugs
- Identify the potential mechanism behind any cardiotoxicities
In the following article, we describe the nonanimal models used in cardiovascular safety pharmacology and some drug targets that have been shown to cause cardiotoxicity.
Why translational cardiovascular safety pharmacology is important
Despite years of preclinical testing, most new drugs fail clinical trial due to inadequate safety or efficacy. As clinical studies are the most expensive stage of the drug development process, failure at this stage adds significant costs to the discovery pipeline.
Cardiotoxicity is responsible for a significant percentage of clinical attrition andis more prevalent during or after Phase II, as compared with Phase I clinical studies.4 Identifying cardiovascular risk early and with high confidence is therefore critical to reducing the cost of drug development.
To achieve this, it is imperative that safety studies use models that can detect meaningful changes in the cardiovascular system. This would help predict cardiotoxicities earlier in the development process and reduce drug development costs in accordance with the "fail early, fail cheap" approach.
Nonanimal cardiovascular safety models
An over-reliance on animal testing is one reason that preclinical safety studies do not accurately predict cardiotoxicities. Species differences can affect the relevance of the data generated by either over or underestimating drug response in humans. The ICH S7A guidelines even acknowledge that cardiotoxicity may not be evident from animal studies alone and encourage alternative testing methods where suitable.3,4 In the next section, we explore some of the alternative testing methods available to researchers in cardiovascular safety pharmacology.
Receptor binding assays
Receptor-binding assays use cell membrane preparations to determine the affinity of a labelled compound in vitro. They can determine binding affinity across a wide range of GPCRs, ion channels, and other potential sites of drug action.7While they cannot characterize the biological function of a compound, receptor binding assays can identify off-target receptors that merit further investigation. Once the affinity for a specific receptor is determined, the biological consequence of receptor binding should be investigated, for example by using ex vivo cardiovascular studies to obtain a cumulative concentration-response curve (CCRC) that determines drug potency and the magnitude of any effect.
Ex vivo cardiovascular studies
Ex vivo cardiovascular studies use human fresh cardiovascular tissues to predict drug safety before clinical trials. These living tissue assays can be used throughout the entire drug discovery pipeline to determine whether a drug modifies cardiac output or by influencing vascular tone, may alter total peripheral resistance.
While not high throughput, ex vivo cardiovascular studies can determine clinically-relevant endpoints that in vivo testing cannot. For example, human resistance arteries can predict the effects of novel compounds on systemic blood pressure: a response usually observed at the tissue or organ level due to differences in receptor expression in blood vessels from different organs.
As systemic total peripheral resistance is primarily influenced by the vascular beds of the skin, gut and skeletal muscles, a common approach is to isolate blood vessels from one or more of these beds and to expose the vessels to the test compound. Subcutaneous arteries from skin samples are most commonly used, being the most readily available from cosmetic procedures.
The arteries are dissected from subcutaneous biopsies and mounted in a wire myograph system to measure contractility. In some instances, perhaps due to information gathered from receptor binding studies, there may be a concern that the test compound could influence pulmonary blood flow. Investigations may therefore take place in both pulmonary and systemic isolated blood vessels to provide an indication of the safety risks in both pulmonary and systemic circulations.
Subcutaneous Resistance Arteries
Use human subcutaneous resistance arteries to assess the effect of your test articles on vascular contractility.
Human tissues vs animal tissues
A key benefit of using human tissues to predict drug safety is their increased clinical translatability compared with animal testing. Animal tissues can be used ex vivo to estimate vascular contractility; however, this has been shown to affect the translatability of generated data. For example,canine subcutaneous arteries have been shown to produce a very different response to 5-HT (serotonin) ex vivo compared with human subcutaneous arteries ex vivo (figure 1).
Figure 1: Canine subcutaneous arteries produce a different response.to 5-HT compared withhuman subcutaneous arteries.
Drug targets commonly associated with cardiotoxicity
Translatability in cardiovascular safety pharmacology is especially important if a compound class has known effects or if the regulators have flagged a specific concern. Good quality preclinical toxicology can be the difference between the regulators approving a compound for clinical testing or requesting more data. Below we have listed drug targets commonly associated with cardiotoxicity that are often investigated thoroughly before human studies.
Relaxin-2 (RLN2)
Relaxin-2 (RLN2) is secreted by the heart and is a powerful vasoconstrictor of ex vivo subcutaneous resistance arteries. It exerts its cardiovascular effects by binding to the relaxin family peptide receptor 1 (RXFP1) and is part of a wider human relaxin peptide family.
VEGF receptor(VEGFR)
Most drugs that inhibit VEGFR (vascular endothelial growth factor [VEGF] receptors) are approved for the treatment of cancers including sunitinib, sorafenib, and pazopanib. However, at clinically therapeutic exposure, these drugs are also associated with a 15–60% incidence of hypertension.8
5-HT2A agonists
The 5-HT2A receptor is expressed on the smooth muscle of human arteries and mediates vasoconstriction. While the link between long-term cardiovascular disease (CVD) and 5-HT2A receptor function is not well established, concerns have been raised about drugs targeting this receptor. Agonism of the 5-HT2A receptor may present a risk of cardiovascular adverse events including:
- ↑ total peripheral resistance (TPR) and blood pressure
- organ-specific effects e.g., ↓ coronary artery perfusion which might increase the risks of angina or myocardial infarction
Serious cardiac events are rare for 5-HT2A agonists currently on the market, but some 5-HT2A agonists and anti-depressants have been shown to affect blood pressure.
5-HT receptors / 5-HT in coronary arteries
This model assesses the effect of your test article on coronary artery vasoconstriction via 5-HT (serotonin) receptors in comparison to 5-HT (serotonin).
5-HT receptors / Denuded coronary arteries
This model assesses the effect of your test article on vasoconstriction of denuded arteries via 5-HT (serotonin) receptors in comparison to 5-HT.
5-HT1B agonists
There appears to be a link between 5-HT1B agonism and rare ischemic events; cardiovascular disease, peripheral artery disease and angina are contraindications for the use of 5-HT1B agonists in migraine treatment. It is therefore advisable to assess the cardiac safety of compounds affecting these receptors before clinical trial. You can learn more about the complicated history, physiology, and pharmacology of 5-HT1B agonists.
5-HT receptors in denuded arteries
This model assesses the effect of your test article on vasoconstriction of denuded arteries via 5-HT (serotonin) receptors, in comparison to 5-HT.
5-HT receptors and 5-HT
This model assesses the effect of your test article on vasoconstriction via 5-HT (serotonin) receptors, or in comparison to 5-HT (serotonin).
5-HT receptors and sumatriptan
This model assesses the effect of your test article on coronary artery tone using sumatriptan as a reference compound.
5-HT2B agonists
The regulators consider stimulation of the 5-HT2B receptor a serious safety concern that may preclude any further preclinical development of a test article. This is because there is an established link between their long-term use and drug-induced valvulopathies. If a drug does display off-target effects, the criteria for clinical advancement depend on a risk-benefit analysis.
Contractile force in human ventricular trabeculae muscle (5-HT receptor)
This assay assesses whether a drug causes an increase in contractile force in human ventricular muscle with 5-HT as a reference compound.
Contractile force in human atrial pectinate muscle (5-HT receptor)
This assay assesses whether a drug causes an increase in contractile force in human atrial muscle with 5-HT as a reference compound.
Conclusion
Translational cardiovascular safety studies are critical to reducing harm and improving the efficiency of drug development. While animal models represent the gold standard of cardiovascular safety assessment, species differences can result in clinical attrition. It is therefore recommended to support animal studies with tests in human cardiovascular tissues.
If you are looking to assess your compounds in cardiovascular tissues but don't know where to start, REPROCELL's human tissue testing experts can help. We can test the effects of your compound on human blood vessels, and even compare drug responses between animal and human tissues to investigate off-target drug effects. You can find examples of our work in our assay catalog or contact us to arrange a custom solution for your research needs.
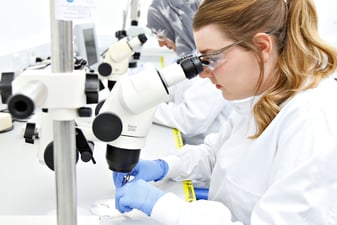
Preclinical and drug discovery CRO
Our contract research services use human living tissues to better predict drug safety and efficacy.
References
- Hentz KL et al. Toxicology Testing and Evaluation in Comprehensive Toxicology (2010).
- Castagne V et al. CNS Safety Pharmacology in Reference Module in Biomedical Sciences (2014).
- European Medical Agency (EMEA). ICH S7A Safety pharmacology studies for human pharmaceuticals EMEA (2001).
- Bhatt S et al. Statistical power analysis of cardiovascular safety pharmacology studies in conscious rats.Journal of Pharmacological and Toxicological Methods 81 pp 128-135 (2016).
- Kenakin TP et al. Safety Pharmacology in A Pharmacology Primer - 5th Edition (2019).
- Faqi AS et al. Nonclinical Safety Assessment of Botanical Products in A Comprehensive Guide to Toxicology in Nonclinical Drug Development - Second Edition (2017).
- Weissman A et al. Drug Discovery Technologies in Comprehensive Medicinal Chemistry II (2007).
- Skinner M et al. The contribution of VEGF signalling to fostamatinib-induced blood pressure elevation. British Journal of Pharmacology 171 pp 2308-2320 (2014).
Editors note: This article was originally published on the 3rd of August 2022 and has since been updated for accuracy and clarity.