Resistance arteries are a group of blood vessels that regulate up to 90% of peripheral vascular resistance.1 There are three types of resistance vessels in humans: precapillary sphincters, arterioles, and small arteries.2 They are important for controlling blood flow to vital organs, such as the heart (coronary arteries) and the brain (cerebral resistance arteries) so are often considered in cardiovascular safety studies.1,3 In the following article, we have summarized the physiology of resistance arteries in health and disease.
Coronary Artery Models
These assays use human coronary arteries to assess the effect of your test article on vasoconstriction.
Subcutaneous Resistance Artery Models
These assays use subcutaneous resistance arteries to assess the effect of your test article on vasoconstriction.
The structure of resistance arteries
The wall of resistance arteries comprises three distinct layers: the adventitia, tunica media, and tunica intima.4 The tunica intima is composed of a subendothelial layer sandwiched between an inner elastic lamina (IEL) and a single layer of endothelial cells.4 This endothelial layer provides a protective barrier between the underlying tissues and the bloodstream.4
The tunica media forms 60-65% of the artery wall and is composed mainly of smooth muscle cells surrounded by an extracellular matrix (ECM).1 This smooth muscle layer forms a significant portion of the media; extending from the tunica intima to the external elastic lamina (EEL).1,4 The adventitia is the outermost layer of the resistance artery wall and is composed mainly of collagen, elastin, and fibroblasts.4
Endothelial cells
Endothelial cells are flat, thin cells that act as the bodies "lining" tissues, providing protection against the external and internal environment. In resistance arteries, endothelial cells form a single-cell layer that protects the underlying connective tissues from the blood flow.4 This endothelium also controls which substances enter and leave the bloodstream.4
However, the main role of the endothelium in these arteries is regulating tone through the synthesis and secretion of vasoactive substances.4 This "vascular endothelial function" is critical for controlling blood pressure and is often disrupted in cardiovascular disease (CVD).4,5 Endothelial cells in resistance arteries are unique in their ability to communicate directly with neighboring smooth muscle cells via holes in the IEL.1
Vascular smooth muscle cells
Vascular smooth muscle cells (VSMCs) make up the smooth muscle fibers in the tunica media. Contraction and relaxation of these fibers cause vasoconstriction and vasodilation respectively.4 Additionally, VSMCs activate endothelial cells during vasoconstriction which triggers endothelial-dependant vasodilation.5 This myoendothelial feedback (MEF) is the primary mechanism for inducing nitric oxide (NO) synthesis and endothelium-derived hyperpolarisation in resistance arteries.5
The function of resistance arteries
Total peripheral resistance (TPR) is an important determinant of blood pressure, and its regulation is a key function of the resistance vasculature.1 While resistance arteries have a maximum lumen diameter of 100 - 350 μm, making them much smaller than other major vessels, even a minor reduction in their size can significantly increase TPR.3,4 This is due to Poiseuille's law, which dictates that resistance to flow (8ηl) is inversely related to the fourth power of the vessel radius (ΔPπr^4) i.e., ΔPπr^4 / 8ηl. If you are interested in finding out more, the following video explains Poiseuille's law in more detail.
Cellular signaling pathways
Resistance artery tone changes in response to biological signals, such as neuronal activity.5 These signals trigger the release of vasoactive substances from the endothelium that activate signaling pathways in VSMC.5 These pathways alter the concentration of calcium ions (Ca2+) in the VSMCs which results in muscle contraction or relaxation.5 An increase in cytosolic Ca2+ activates Protein Kinase-C (PKC), inducing smooth muscle contraction and vasoconstriction.5 Conversely, a decrease in cytosolic Ca2+ causes smooth muscle relaxation and vasodilation.5
Endothelin-1 (EDN1)
Endothelin-1 (ET-1) is an important vasoactive stimulant in resistance arteries. It triggers vasodilation and cell proliferation through two VSMC receptors: endothelin type A (ETA) and endothelin type B (ETB).6 ET-1 causes direct vasodilation and cell proliferation through the activation of receptor ETA.6 Conversely, activation of receptor ETB causes indirect vasodilation by stimulating the release of NO and prostacyclin (PGI2).6
Nitric oxide (NO)
NO is another crucial vasoactive substance that regulates resistance artery tone through cellular signaling pathways. It is synthesized and secreted by endothelial cells in response to sheer stress and calcium-mobilizing agents.5,6 NO is a potent vasodilator that has anti-proliferative effects on VSMCs; it also blocks leukocyte adhesion and thrombocyte aggregation.6
Vasodilation of ischemic resistance arteries via NO donors
Use ischemic resistance arteries to assess the effect of your test article on vasodilation via the NO donor SNP.
Vasodilation of subcutaneous resistance arteries via NO donors
Assess the effect of your test article to induce vasodilation via endothelium-independent mechanisms.
Ion Transport
As intracellular pH is an important determinant of muscle tone, ion transport is critical for regulating vasoconstriction and dilation.5 Key ion transporters in VSMCs include the Na+/bicarbonate transporter (SLC4A10), Na+/Ca+ exchanger (SLC8A1), and Na+/H+ exchanger (SLC9A1).6 Several ion channels are involved in regulating the currents that change the membrane potential and alter the intracellular calcium concentration, [Ca2+]i, which we have summarized in table 1.
Hyperpolarising outward current (K+) | Depolarising inward current (Ca2+) |
Voltage-gated (KV) | Ca2+-activated Cl- |
Ca2+ activated (BKCa, IKCa, SKCa) | Voltage-operated Ca2+ |
ATP Sensitive (KATP) | TRP-like non-selective cation-currents |
Inward Rectifying (KIR) |
Table 1: Summary of currents that control membrane potential (VM) in the smooth muscle of resistance arteries. Information from Sancho et al., 2020.5
Protein Kinase-C (PKC)
Upon activation, the intracellular messenger PKC mediates VSMC contraction through actin polymerization and cytoskeletal re-organization.7 While its role is most significant in cerebral resistance arteries, it also mediates VSMC contraction in coronary and subcutaneous resistance arteries.7 There are several mechanisms PKC is thought to mediate myogenic contraction listed in table 2.
Action used by PKC to mediate myogenic contraction | Level of change |
Reducing the concentration of G-actin | Post-transcriptional |
Reorganization of the actin cytoskeleton | Post-transcriptional |
Increasing paxillin and HSP27 phosphorylation | Post-transcriptional |
Modulating structural protein genes e.g., type IV collagen, fibronectin | Genetic |
Changing expression of Regulator of G-protein Signalling (RGS2) | Genetic |
Table 2: Table summarizing how PKC modulates myogenic contraction. Information from Ringvold et al., 2017.4,7
Hypertension and resistance arteries
Resistance arteries contribute significantly to the development of hypertension and its associated complications.6 Approximately a third of UK adults are hypertensive, and the condition affects over one billion people worldwide.8,9 It is a major cause of premature death globally and has a complex etiology with multiple environmental and genetic factors involved.1,9
In hypertensive patients, resistance arteries undergo functional and structural changes due to abnormal extracellular stimuli and cellular signaling pathways.4,6 However, the specific molecular mechanisms behind these alterations remain to be defined; several endogenous messengers are thought to be involved including cytokines, growth factors, and vasoconstrictors.5,6
Functional changes observed in hypertension
Increased constriction is commonly observed in the resistance arteries of hypertensive patients.4 This vasoconstriction has a significant effect on blood pressure, regardless of whether downstream blood vessels are affected or not.1 Endothelial and smooth muscle dysfunction in resistance vasculature is a hallmark of hypertension observed in many patients.3,6 These cellular changes may be the result of altered ECM-integrin interactions.3,6 For example, endothelium-dependant relaxation is usually triggered by αvβ3 activation, yet these integrins may not be available for binding in hypertensive patients.6 Observed dysfunction may also extend to adipocytes, fibroblasts, and immune cells.3
Structural changes observed in hypertension
Structural changes to resistance arteries in hypertrophic patients include hypertrophic and eutrophic vascular remodeling.3 Both decrease lumen diameter and increase the arterial media/lumen (M/L) ratio, but there are important differences between them.3
Hypertrophic remodeling is only observed in patients with chronic/severe disease and is characterized by the enlargement of VSMCs.3,4,10 On the other hand, eutrophic remodeling is observed in patients with mild-severe hypertension but does not cause hypertrophy; instead, the smooth muscle cells are rearranged around a smaller lumen and outer diameter.4 Both types of remodeling amplify any functional changes further, creating a negative feedback loop that causes increasing TPR over time.3
Benefits of testing drugs in human resistance arteries
Essential hypertension is a complex disease that requires a multifactorial approach to treatment and research.1 Unfortunately, many of the preclinical models used to study this disease are limited. For instance, in vitro models fail to mimic disease complexity, and the relevance of animal models varies depending on the species.1 As a result, data from these models often lack clinical translation compared to experiments using human tissues (figure 1).
A | ![]() |
B | ![]() |
Figure 1: Example of donated resistance arteries used in human tissue experiments. A, Human Subcutaneous resistance arteries. B, Human Human Coronary Arteries.
On the other hand, human tissue testing provides more relevant drug efficacy and safety data. For example, when pharmacologists were developing AMG337, canine resistance arteries demonstrated a reduced relaxation response compared with human resistance arteries (figure 2).11 As the safety profile of the drug had only been tested in animals, researchers were not aware of this significant species difference. When clinical trials began, human subjects experienced headaches in response to AMG337 that had not been anticipated preclinically.11 By going back to the lab and testing the drug in human resistance arteries ex vivo, researchers identified this species difference and were able to investigate the mechanism behind it. If you would like to find out more about human tissue testing, we have summarised everything you need to know in this blog.
Figure 2: Relaxation response of AMG 337 (shown as % relaxation) in precontracted (A) dog and (B) human blood vessels. Blue, Vehicle; Black, AMG 337. Adapted from Amouzadeh et al., 2019.11
Conclusion
The resistance vasculature is a key regulator of peripheral resistance and blood pressure. Vascular tone is controlled by several mechanisms, including myoendothelial feedback, endothelium-derived hyperpolarisation, and neural control. The structure and function of these arteries are changed in hypertension, leading to an increase in arterial pressure and TPR.
If you are looking to assess the effect of your test compounds on resistance arteries, ex vivo testing in human resistance arteries offers a translational alternative to animal models. Find out how REPROCELL can test your drugs in living human resistance arteries below.
Coronary Artery Models
These assays use human coronary arteries to assess the effect of your test article on vasoconstriction.
Subcutaneous Resistance Artery Models
These assays use subcutaneous resistance arteries to assess the effect of your test article on vasoconstriction.
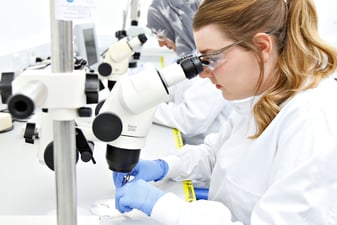
Preclinical and drug discovery CRO
Our contract research services use human living tissues to better predict drug safety and efficacy.
References
- Lee R et al. Vascular structural and functional changes: their association with causality in hypertension: models, remodeling and relevance. Hypertension Research (2017).
- Prasad K. Structure and Function of Various Vascular beds. Textbook of Angiology (2000).
- Touyz RM et al. Arterial Hypertension. Muscle: Fundamental Biology and Mechanisms of Disease (2022).
- Schiffrin EL et al. Remodeling of Resistance Arteries in Hypertension and Effects of Anti-Hypertensive Treatment. American Journal of Hypertension (2005).
- Sancho M et al. Ion channels and Calcium Signaling in the Microcirculation. Current Topics in Membranes (2020).
- Gong et al. Hypertension Pharmacogenomics. Handbook of Pharmacogenomics and Stratified Medicine (2014).
- Ringvold HC et al. Vascular Pharmacology. Advances in Pharmacology (2017).
- National Health Service (NHS). High blood pressure (hypertension). NHS (2019).
-
World Health Organisation (WHO). Hypertension. WHO (2022).
-
Gradman AH et al. Angiotensin-Converting Enzyme Inhibitors. Comprehensive Hypertension (2007).
- Amouzadeh et al. Clinical Implications and Translation of an Off-Target Pharmacology Profiling Hit: Adenosine Uptake Inhibition In Vitro. Oncol. 12, 1296–1304 (2019).