How to mass culture induced pluripotent stem cells (iPSCs)
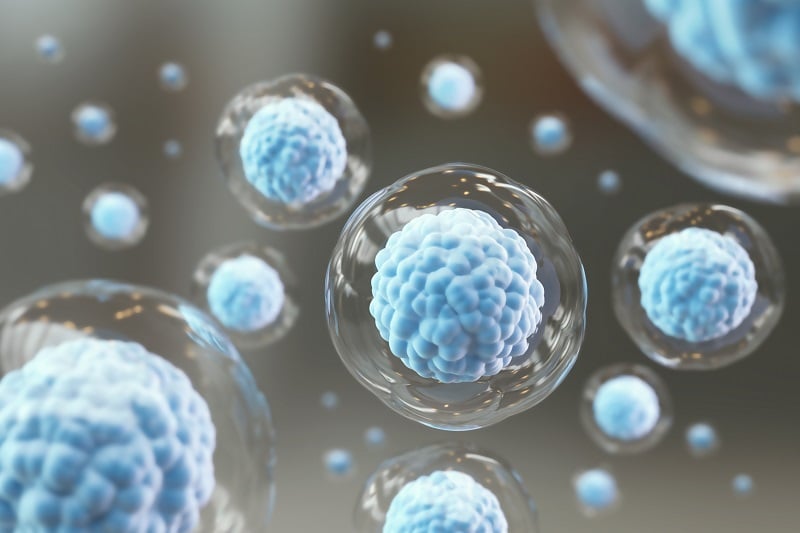
Induced pluripotent stem cells (iPSCs) have the potential to revolutionize the fields of drug discovery, disease modelling, personalized medicine and regenerative medicine [1]. iPSCs have the capacity for continuous self-renewal and differentiation into several different cell types. At the same time, they also do not face many of the ethical challenges faced by human embryonic stem cells [2].
In order to fully realize the potential of iPSCs for many of the above applications, there is a requirement to generate a vast number of cells; hence, an effective method of mass culture of iPSCs is highly sought after by iPSC researchers.
Why is iPSC Mass Culture important?
It is estimated that to replace disease-induced loss of hepatocytes, pancreatic β-cells, or cardiomyocytes, approximately 1–10×109 functional cells per patient are required [3]. For production of iPSC derived blood “in vitro blood”, even higher amounts (approximately 2.5×1012 red blood cells) are required per patient in transfusion medicine [4].
Moreover, for drug discovery applications like high-throughput screening (HTS), many iPSCs are needed because iPSC-derived cells are seeded in multiwell plates and handled via automated systems. Such systems often achieve throughput rates of the order of 10,000 compounds per assay per day [5] making scale-up of iPSC production a major barrier
iPSC cultures are conventionally carried out in 2D cell culture plates, dishes or flasks with cells adhering to the matrix-covered surface of culture plates or flasks. 2D cell culture typically relies on static culture conditions known to induce the formation of undesired gradients, including media components, metabolic waste products, paracrine factors, and gases [6].
Therefore, with some exceptions, most 2D iPSC culture techniques require almost daily media changes, which is quite cumbersome. To generate larger numbers of cells, ‘scale-out’ of the 2D approach is often used by multiplying culture dishes or by using multi-layered flasks; hence, the 2D approach is relatively cost-, space-, and labor-intensive [3].
Download our ABLE Biott Brochure →
In contrast, using a small benchtop 3D suspension bioreactor system such as the ABLE Biott bioreactor allows process ‘scale-up’ without ‘scale-out’ [5]. This enables the mass culture of the same amount of iPSCs using far fewer plates and culture media, without the necessity of external matrix supplementation and with less frequent feeding.
The dynamic suspension cultures facilitated by the ABLE Biott bioreactors promotes the control of iPSC aggregation, avoids the formation of gradients by supporting homogeneous distribution of cell culture components, and improves the transfer of gases and nutrients into cells/aggregates, hence facilitating mass iPSC cultures at relatively high cell density [7]. This helps cut down on the cost of cell culture media and matrix, while saving space in the lab and reducing hands-on time. For example, one 30ml ABLE Biott bioreactor enables expansion for up to 5 × 107 cells; approximately equivalent to cell yields from ten 10 cm culture dishes or ten 6-well plates.
The growth of iPSCs in 3D spheroid suspension culture closely resembles embryoid body structures naturally formed by embryonic stem cells. It is considered that cultivation in suspension culture based on matrix-free cell-only aggregates may be a closer match to human embryonic stem cells’ “in situ niche” [3] i.e., equivalent to the inner cell mass of the early human embryo at the blastocyst stage from which these cells originate [8].
Human iPSCs (cell line 1231A3) grown in StemFit™ Medium, demonstrating the consistency of spheroid sizes after 4 days cultivation in the ABLE 3D Disposable Bioreactor at 40 rpm.
How to mass culture pluripotent stem cells
ABLE Bioreactors come as a plug-and play system, which includes a controller and motor, a stir system base and disposable bioreactor vessels of various capacities i.e. 5 ml, 30 ml, 100 ml and 500 ml. Multiple disposable bioreactor vessels can be set up on a single plate at one time. In order to scale up your iPSC cultures depending upon the cell numbers needed, one can:
- Culture iPSCs to confluency in a 10 cm plate, and then transfer them into the smallest culture vessel size. For this you will require:
Note: The 5 ml ABLE culture vessel can culture 5×106 iPSCs – the same as one 10 cm plate. The delta-shaped impeller minimizes cell collision during culture, by ensuring that cells flow laterally at a constant rate.
- Once your iPSCs are confluent, move them into a larger culture vessel. For this you will require:
- ABLE 30 ml culture vessel
- ABLE 100 ml culture vessel
- 30 ml/100 ml magnetic stirrer base
- Bioreactor control system and motor (bought previously; see above)
Note: The 30 ml ABLE culture vessel can culture 5×107 iPSCs, the equivalent of ten 10cm plates. The ABLE 100ml can culture 2×108 cells per vessel (or more), equivalent to about forty 10 cm plates.
- Finally, your cells can be transferred into the largest bioreactor culture vessel. For this step, you will require:
- ABLE 500 ml culture vessel
- Any suitable lab magnetic stirrer (heat-free)
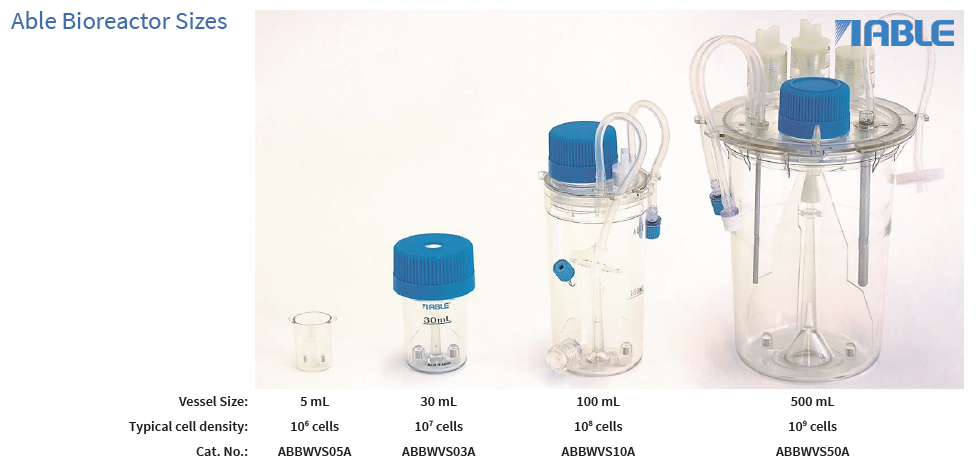
Cost-efficient alternative to the scale-out approach
Scale-up of iPSC cultures using the ABLE Biott 3D suspension bioreactor offers a more cost-efficient, space-saving, and labor-saving alternative to the scale-out approach with 2D cultures.
To fins out more, download our brochure.
For further technical and ordering queries, please contact info-emea@reprocell.com.
References
- Robinton et al. The promise of induced pluripotent stem cells in research and therapy. Nature 481:7381 (2012)
- Welin. Ethical issues in human embryonic stem cell research. Acta Obstet Gynecol Scand 81 (2002)
- Kropp et al. Progress and challenges in large-scale expansion of human pluripotent stem cells.Process biochemistry 59 (2017)
- Migliaccio et al. The potential of stem cells as an in vitro source of red blood cells for transfusion. Cell Stem Cell 10: 2 (2012)
- Eaker et al. Concise review: guidance in developing commercializable autologous/patient-specific cell therapy manufacturing. Stem Cells Translational Medicine 2 (2013)
- Want et al. Large-scale expansion and exploitation of pluripotent stem cells for regenerative medicine purposes: beyond the T flask. Med. 7 (2012)
- Zweigerdt. Large scale production of stem cells and their derivatives. Adv. Biochem. Eng. Biotechnol., 114 (2009)
- Blakeley et al. Defining the three cell lineages of the human blastocyst by single-cell RNA-seq. Development 142 (2015)
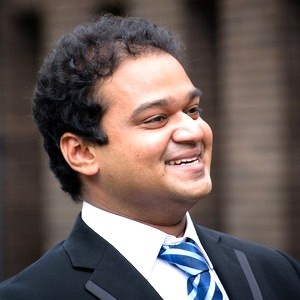
Author
Chirag Tawde, MSc
Sales Director EMEA at REPROCELL
Chirag is responsible for managing sales and business development activity in EMEA for REPROCELL. He loves smashing sales targets, driving on scenic country roads, and exploring new cities. You can contact him on
LinkedIn.
Subscribe to receive updates from REPROCELL
Tagged
Stem cell and drug discovery scientists around the world are using our services and products in their preclinical and clinical research.
Subjects we write about
- 3D cell culture
- Cell culture
- Central Lab Services
- Clinical capabilities
- Disease modeling
- Drug discovery
- Gene editing
- Genomic services
- GMP
- Human tissue samples
- Human tissue testing
- IBD
- Life sciences
- Neurons
- Oligonucleotide synthesis
- Precision medicine
- Regenerative medicine
- Respiratory disease
- Skin disease
- Stem cells