Pluripotent Potential for Clinical Application
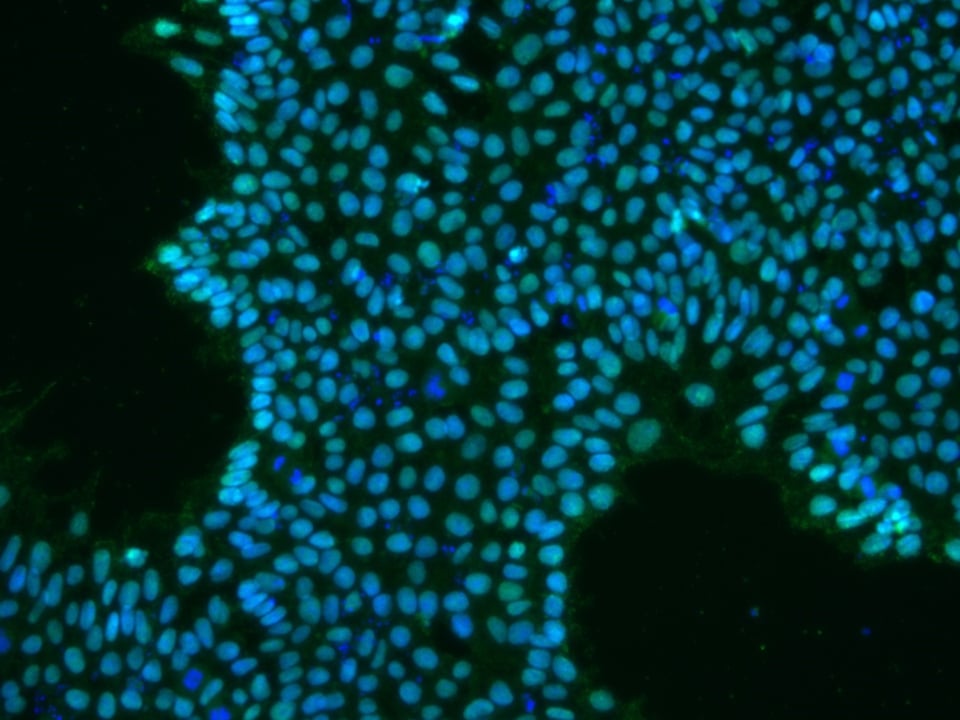
Since their development in the mid-2000s, the versatile nature of induced Pluripotent Stem Cells (iPSCs) has unlocked the potential of curative approaches instead of symptom-reactive treatments. In particular, fields which deal with genetic disorders and regenerative therapies would benefit from this. The advancement of iPSC technology compliments the advent of personalized medicine, allowing for a future where individuals could be treated using autologous iPSCs.
Further research is needed before iPSCs are considered clinical-grade. REPROCELL can help you to become involved at the forefront of this research. Our StemRNA 3rd Gen Reprogramming Kit allows for non-modified RNA and microRNA cellular reprogramming using human fibroblasts, urinary progenitor cells (UPCs), and blood-derived endothelial progenitor cells (EPCs). From these, primary iPSC colonies will be ready within 10-14 days.
What are induced pluripotent stem cells?
In 2007, Takahashi et al [1] published their pioneering technique for developing human iPSCs (hiPSCs). Following retroviral introduction of transgenes into human dermal fibroblasts, they were able to reproduce the morphology, growth patterns, and marker genes consistent with Embryonic Stem Cells (ESCs). The four transcription factors essential for this were Oct4, Sox2, c-Myc, and Klf4 and, following cultivation of embryoid bodies (EBs), it was possible to derive all three germ layers [1].
A little over a decade later, iPSC reprogramming techniques have expanded due to new methods, biomarker identification, and clinical considerations.
Figure 1: iPSCs expressing OCT4 (green)
Reprogramming Improvements
-
RNA-based reprogramming allows iPSCs to be created without the risk of unregulated transgene-genome integration [2]. iPSCs established in this way also display gene expression patterns similar to ESCs. Nakajima et al used REPROCELL’s StemRNA-NM Reprogramming kit and our NutriStem medium to reprogram marmoset fibroblasts. Cells with iPSC characteristics were successfully reproduced by using transfected mRNAs encoding human transcription factors Oct4, Sox2, Klf4, c-Myc, Nanog, and Lin28 [2], thus eliminating the transgene risk and improving iPSC stability.
-
Hypoxic conditions have been reported to increase production of hiPSCs [3]. Using ReproStem as a growth medium to maintain their hiPSCs, Sugimoto et al’s undifferentiated colonies more than doubled when grown in 5% oxygen as opposed to normoxic conditions. Transcription factor expression also showed a correlated increase [3]. In particular, OCT4 increased nine-fold, while expression of SOX2 and NANOG doubled (Figure 2). A way to produce iPSCs on a large scale is necessary for their successful integration into existing healthcare practices; using hypoxic conditions would be one way to increase their chances for this.
Figure 2: The relative expression levels of pluripotency markers in 20% oxygen vs. 5% oxygen conditions [3]
- Another method of increasing production would be to use stirred-tank bioreactors: this is a method proved by Kropp et al to improve cell yield by 47%[4]. It also allows 3D growth of cells as opposed to the traditional 2D culturing, creating both larger volumes of cells and increasing culture-monitoring capabilities. Further research is needed into finding how similar the gene expression of these iPSCs is when compared with traditional 2D-cultured iPSCs.
-
Similarly, it has been found that microRNAs (miRs) play an important role in regulating cell fate, pluripotency, and cell proliferation in ESCs [5]. Expression of a certain cluster of miRs (miR302/367) primes cells for reprogramming; this results in more efficient generation of iPSCs by at least two orders of magnitude, without the instability associated with the ectopic introduction of proteins [6].
-
Although iPSCs can be autologous, introducing synthesised mRNAs to cells still provokes an immune response. Immune ablation using three factors (E3, K3, and B18R) reduces cell response to foreign RNA, thus preventing cell death and making iPSCs more likely to proliferate. Using vaccinia virus mRNAs to inhibit interferon production is more effective and reliable than other methods using small interfering RNA (siRNA) or modified RNA [7].
-
REPROCELL’s StemRNA 3rd Gen Reprogramming kit uses both miRNA302/367 and immune ablation technology, increasing the reprogramming capability by priming cells for reprogramming and increasing mRNA stability. Importantly, it can also derive iPSCs from UPCs, as well as peripheral-blood derived endothelial cells. This combination of increased reprogramming capabilities, immune ablation, and low-invasion collection of progenitor cells increases applicability of iPSC reprogramming to the clinical world.
New Biomarker Discoveries
-
By identifying biomarkers which predict differentiation potential, appropriate cell lines can be selected for specific purposes. Ohasi et al identified PF4, whose protein is part of the CXC chemokine family, as a biomarker which indicated how well cardiac iPSCs differentiated and proliferated [8]. For their cap analysis of gene expression (CAGE), they maintained the hiPSC lines with REPROCELL’s Primate ES Cell Medium. They found higher PF4 levels correlated with higher differentiation potential, even when PF4 production was artificially stimulated. This marker could be used as a powerful tool for quality control in iPSC production.
-
More specifically, biomarkers are essential to identify stepwise differentiation of cells for tissue-specific function. Matsui et al, through cell culture using REPROCELL’s Primate ES Cell Medium, found that AQP1-GFP, from the aquaporin family, could be used as a cholangiocyte progenitor marker [9]. In particular, the ability to remodel cholangiocytes would be essential in helping people with congenital biliary disorders, allowing further study into differentiation mechanisms and ductal plate malformations.
Clinical Applications of iPSCs
-
Okada et al investigated hiPSC application with regard to treating familial hypercholesterolemia [10]. Using Nanog sourced from REPROCELL in conjunction with conventional CRISPR/Cas9 techniques, they were able to improve Low Density Lipoprotein (LDL) uptake in edited cells. They were able to derive these iPSCs from the patient’s own peripheral lymphocytes. During testing, LDL uptake increased with little immune impact. This low-invasion method for producing iPSCs opens the future potential for iPSCs to be derived via primary and secondary care services at point of patient contact.
- Transplant rejection is a huge obstacle to overcome for medicine. The safe production of autologous tissue would have an immediate positive impact. Although almost all somatic cell types can be derived from iPSCs, multicellular frameworks are complex. Hamanaka et al found that, although they were unable to produce organs from murine iPSCs alone, they were able to generate functional vascular tissue via blastocyst complementation [11]. While iPSC-derived organ replacement is a long way from clinical reality, complex, functional tissue growth is indeed possible for tissue modelling and preclinical drug testing.
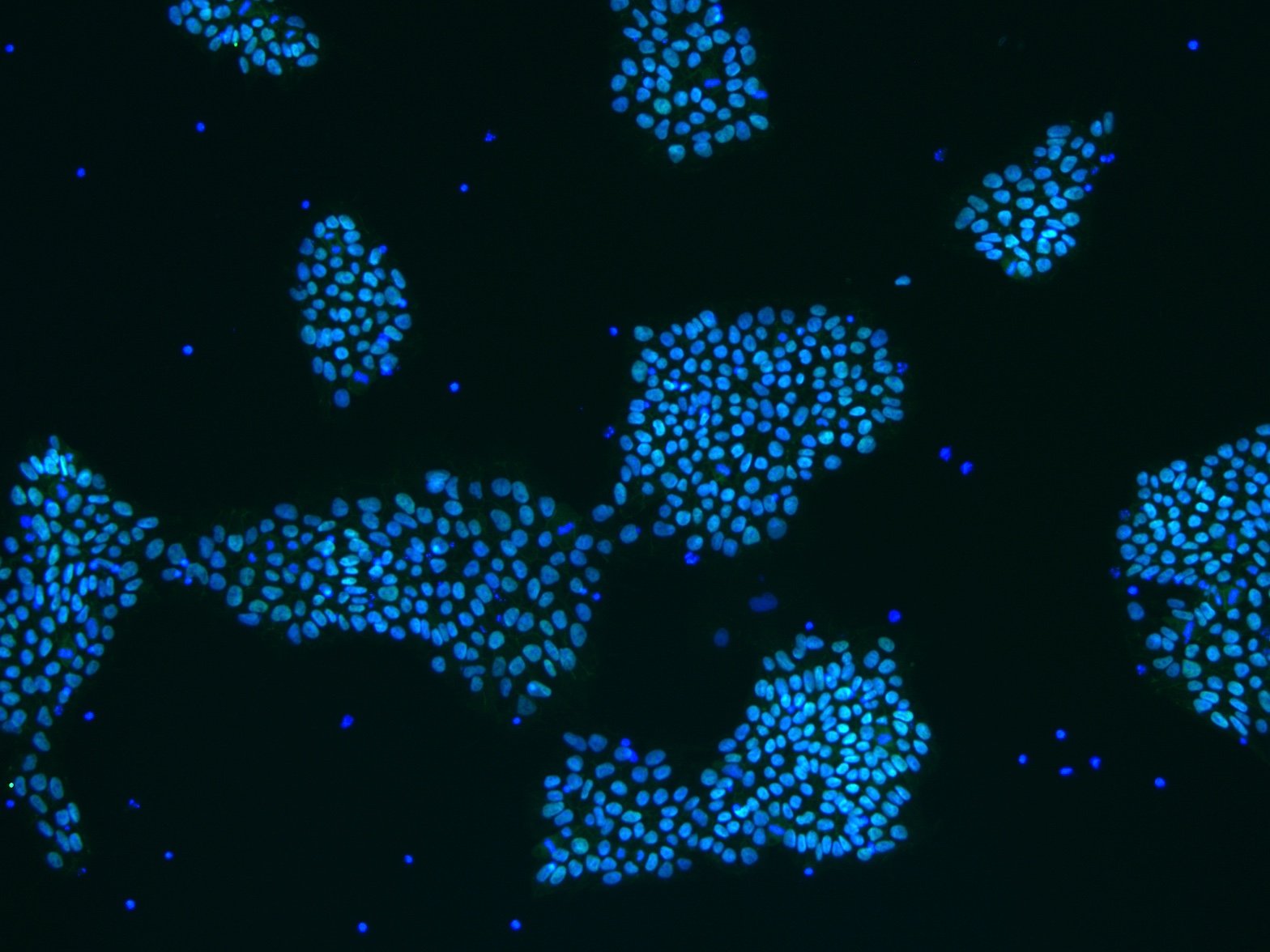
REPROCELL offers a complete portfolio of services for the reprogramming and differentiation of high-quality iPSCs.
The Future of Stem Cell Research
In a decade of iPSCs development, new methods have been found to reduce risk, increase production, identify new biomarkers, improve quality control, and increase successful applications of hiPSCs. Generating clinically relevant iPSCs opens new horizons towards developing life-saving regenerative therapies, pre-clinical drug testing, and personalized medicine.
References
- Takahashi et al. Induction of Pluripotent Stem Cells from Adult Human Fibroblasts by Defined Factors. Cell 5:131 (2007)
- Nakajima et al. Establishment of induced pluripotent stem cells from common marmoset fibroblasts by RNA-based reprogramming. Biochemical and Biophysical Research Communications 4:515 (2019)
- Sugimoto et al. Effects of hypoxia inducible factors on pluripotency in human iPS cells. Microscopy Research & Technique 7:81 (2018)
- Kropp et al. Impact of Feeding Strategies on the Scalable Expansion of Human Pluripotent Stem Cells in Single-Use Stirred Tank Bioreactors. Stem Cells Translational Medicine 10:5 (2016)
- Lipchina et al. The expanding role of miR-302-367 in pluripotency and reprogramming. Cell Cycle 8:11 (2012)
- Anokye-Danso et al. Highly Efficient miRNA-Mediated Reprogramming of Mouse and Human Somatic Cells to Pluripotency. Cell Stem Cell 4:8 (2011)
- Poleganov et al. Efficient Reprogramming of Human Fibroblasts and Blood-Derived Endothelial Progenitor Cells Using Nonmodified RNA for Reprogramming and Immune Evasion. Human Gene Therapy 11:26 (2015)
- Ohasi et al. CXCL4/PF4 is a predictive biomarker of cardiac differentiation potential of human induced pluripotent stem cells.Scientific Reports 9:4638 (2019)
- Matsui et al. Differentiation and isolation of iPSC-derived remodeling ductal plate-like cells by use of an AQP1-GFP report human iPSC line. Stem Cell Research 35 (2019)
- Okada et al. Function and Immunogenicity of Gene-corrected iPSC-derived Hepatocyte-Like Cells in Restoring Low Density Lipoprotein Uptake in Homozygous Familial Hypercholesterolemia. Scientific Reports 9:4695 (2019)
- Hamanaka et al. Generation of Vascular Endothelial Cells and Hematopoietic Cells by Blastocyst Complementation. Stem Cell Reports 4:11 (2018)
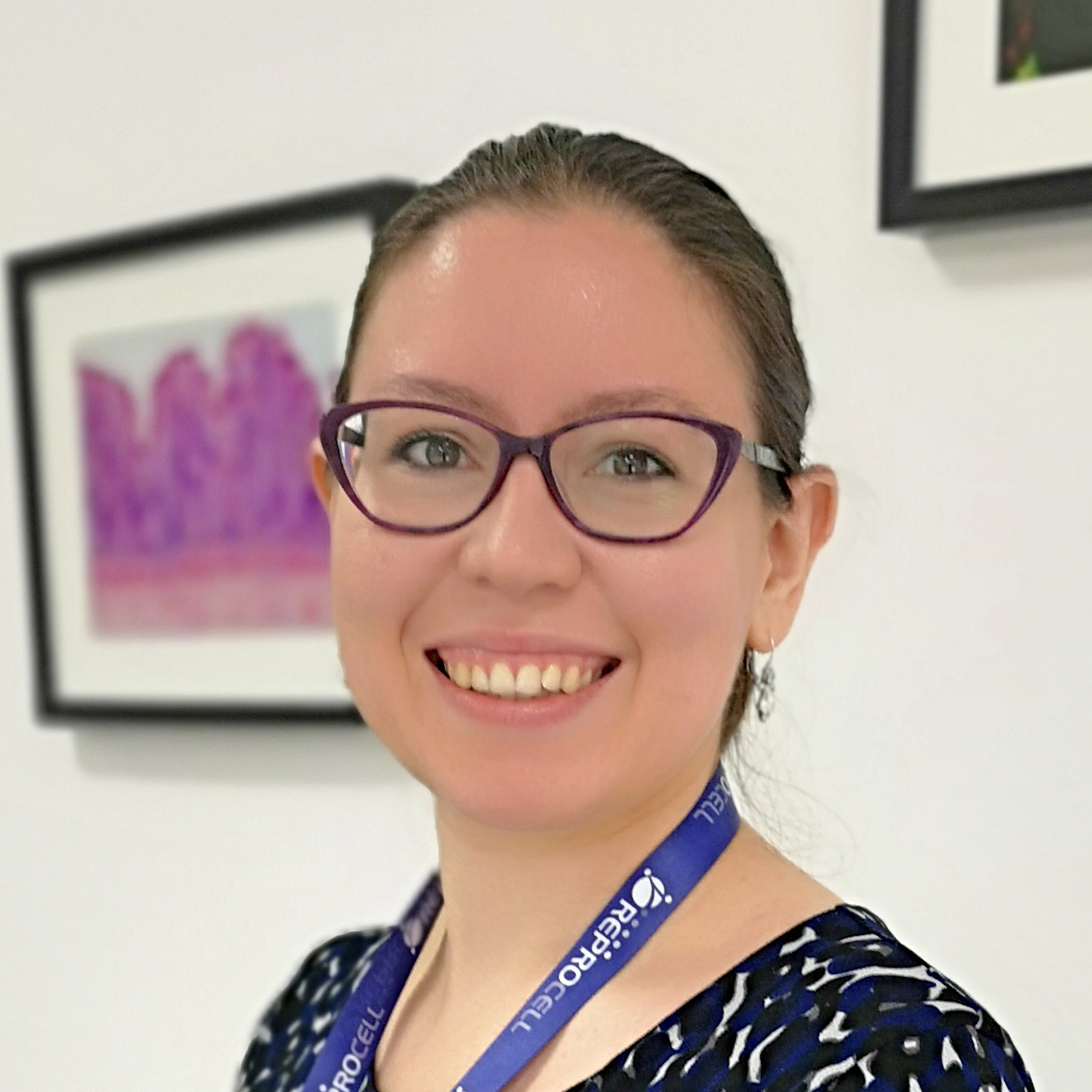
Author
Jennifer McCavitt
Subscribe to receive updates from REPROCELL
Tagged
Stem cell and drug discovery scientists around the world are using REPROCELL’s services and products in their preclinical and clinical research.