Recombinant human vitronectin protein
QK120
Brand: Qkine
Vitronectin protein is widely used in stem cell culture. It provides a defined environment that supports the maintenance of pluripotency and is suitable for feeder-free culture, expansion, differentiation, and reprogramming of stem cells.
Qkine vitronectin is a high-purity animal origin-free recombinant protein with a molecular weight of 47.8 kDa. It is carrier-free and protein tag-free, ensuring exceptional lot-to-lot consistency and compatibility with translational studies and iPSC-based model production for screening. Qkine recombinant vitronectin protein is ultra high-purity with exceptionally low residual endotoxins making it suitable for the reproducible culture of stem cells, primary cells, organoids, and use in sensitive neural differentiations.
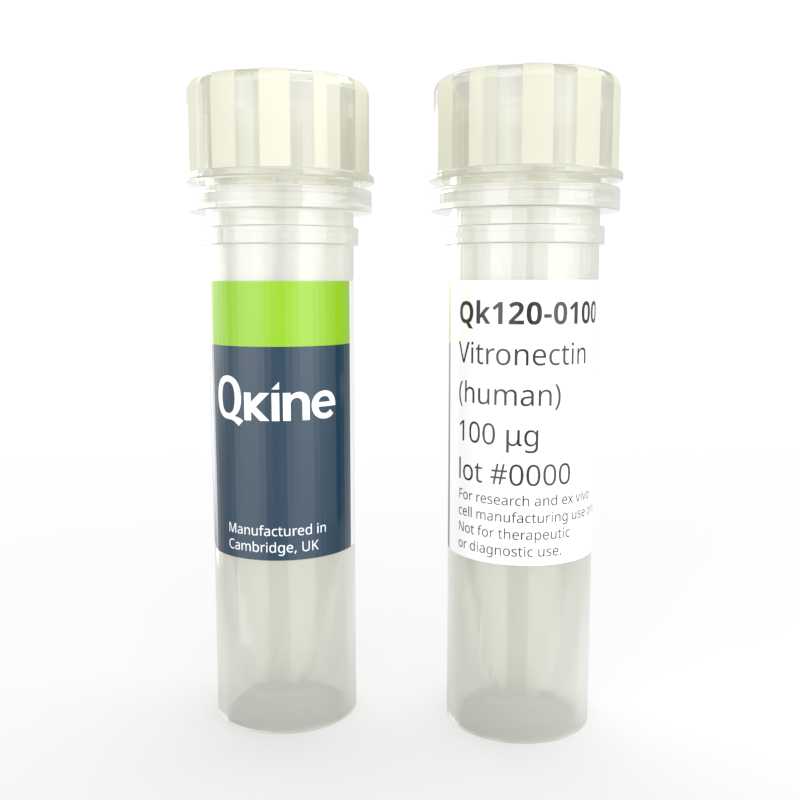
Currency:
Product name | Catalog number | Pack size | Price | Price (USD) | Price (GBP) | Price (EUR) |
---|---|---|---|---|---|---|
Recombinant human vitronectin protein, 500 µg | QK120-0500 | 500 µg | (select above) | $ 150.00 | £ 110.00 | € 129.00 |
Recombinant human vitronectin protein, 5000 µg | QK120-5000 | 5000 µg | (select above) | $ 620.00 | £ 460.00 | € 538.00 |
Note: prices shown do not include shipping and handling charges.
Qkine company name and logo are the property of Qkine Ltd. UK.
Alternative protein names
VTN-N
S-protein
Serum-spreading factor, V75
Species reactivity
human
species similarity:
mouse – 74%
rat – 76%
porcine – 74%
bovine – 72%
Frequently used together with:
- Recombinant FGF2-G3 (145 aa) protein (QK052)
- Recombinant FGF2-G3 (154aa) protein (QK053)
- Recombinant human/bovine/porcine TGF-β1 PLUS™ protein (QK010)
- Recombinant human TGF-β3 protein (QK054)
- Recombinant human VEGF 165 protein (QK048)
- Recombinant human PDGF-BB protein (QK044)
- Recombinant human IGF-1 protein (QK047)
- Recombinant human PDGF-AA protein (QK043)
Summary
- Highly pure recombinant human vitronectin protein (truncated) (UniProt number: P04004)
- >95%, by SDS-PAGE quantitative densitometry
- Source: Expressed in E. coli
- 47.8 kDa, monomer
- Animal origin-free (AOF) and carrier protein-free
- Manufactured in Cambridge, UK
- Lyophilized from PBS, mannitol and TCEP
Resuspend in sterile-filtered water at 1 mg/ml, add carrier protein if desired, and store frozen at -20°C or -80°C
Featured applications
- Maintenance and expansion of human iPSC, ESC and primary cells
- Directed differentiation of iPSCs towards multiple lineages (tri lineage differentiation protocols)
- Promotion of cell adhesion and migration
- Generation of tissue-specific organoids
- Stimulation of angiogenesis and vascular network development
- Fully defined, animal-free protocols for translational studies and preclinical stem cell production

Imaging of colonies grown on Qkine vitronectin coated plates in E8-like media retained their highly preserved morphological appearance. (A) after initial seeding and 3 days in culture; (B) After 1 passages and 7 days in culture; (C) After 3 passages and 14 days in culture (scale bar = 150µm). 6-well plates were coated with 5 µg/ml Qk120 vitronectin protein lot #204660.
Further quality assays
- Mass spectrometry, single species with the expected mass
- Endotoxin: <0.005 EU/μg protein (below the level of detection)
- Recovery from stock vial: >95%
Preparation of cell culture plates with Qkine ultra-high quality vitronectin (Qk120)
- Briefly centrifuge vial containing vitronectin Qk120 to ensure all lyophilized protein is collected at the bottom of the vial.
- Resuspend in 500 ml of MilliQ water to make a 1 mg/ml stock and lightly agitate the tube to ensure everything has fully reconstituted.
- Per 6-well plate required, dilute 30 µl of the 1 mg/ml in 6 ml of phosphate buffered saline (PBS) without MgCl and CaCl to make 5 µg/ml solution.
- Coat each well of 6-well plate with 1 ml per well of 5 µg/ml vitronectin for at least two hours at 37°C.
- Adjust volume of 5 µg/ml vitronectin per well for different area plate clusters.
- 12-well plate add 500 µl
- 24-well plate add 250 µl
- 96-well plate add 100 µl
- Aliquot the remaining resuspended 1 mg/ml vitronectin into appropriately sized single use aliquots and store at -80°C.
Protein background
In tissue engineering and regenerative medicine, vitronectin-coated scaffolds and biomaterials enhance cell attachment, proliferation, and differentiation, contributing to the development of functional tissue constructs [15-16]. Additionally, vitronectin is used to prepare cells for cell therapy, ensuring their viability and functionality upon transplantation.
Vitronectin is particularly important for optimizing culture conditions in the manufacturing of serum-free media, compensating for the lack of adhesion-promoting factors typically provided by serum and supporting cell growth under defined conditions [7, 17]. The use of recombinant vitronectin protein ensures batch-to-batch consistency, which is critical for reproducibility in experimental and clinical applications.
Structurally, vitronectin is a multifunctional glycoprotein with a molecular weight of approximately 75 kDa. It comprises several domains, including the somatomedin B domain at the N-terminal, which is important for binding to the urokinase receptor and stabilizing the inactive form of plasminogen activator inhibitor-1 (PAI-1). The RCL (Ricinus communis agglutinin-like) domain is crucial for heparin binding, while the hemopexin-like repeats facilitate interactions with integrins and other ECM components [2,6,18]. Vitronectin can undergo conformational changes that expose or hide specific binding sites, enabling it to interact with a wide range of ligands and receptors.
In summary, Vitronectin plays a crucial role in various applications, particularly in stem cell and organoid applications, by providing a defined and supportive environment for stem cell maintenance, differentiation, and reprogramming. Its use enhances the reproducibility and efficiency of several cell cultures, making it a valuable tool in both research and therapeutic contexts.
Background references
- Schvartz, I., Seger, D. & Shaltiel, S. Vitronectin. The International Journal of Biochemistry & Cell Biology 31, 539–544 (1999).
- Feldinghabermann, B. & Cheresh, D. Vitronectin and its receptors. Current Opinion in Cell Biology 5, 864–868 (1993).
- Hurt, E. M. et al. Identification of Vitronectin as an Extrinsic Inducer of Cancer Stem Cell Differentiation and Tumor Formation. Stem Cells 28, 390–398 (2010).
- Jang, Y.-C., Tsou, R., Gibran, N. S. & Isik, F. F. Vitronectin deficiency is associated with increased wound fibrinolysis and decreased microvascular angiogenesis in mice. Surgery 127, 696–704 (2000).
- Preissner, K. T. The role of vitronectin as multifunctional regulator in the hemostatic and immune systems. Blut 59, 419–431 (1989).
- Madsen, C. D. & Sidenius, N. The interaction between urokinase receptor and vitronectin in cell adhesion and signalling. European Journal of Cell Biology 87, 617–629 (2008).
- Chen, G. et al. Chemically defined conditions for human iPSC derivation and culture. Nat Methods 8, 424–429 (2011).
- Hasanuzzaman, M., Kutner, R., Agha-Mohammadi, S., Reiser, J. & Sehgal, I. A doxycycline-inducible urokinase receptor (uPAR) upregulates uPAR activities including resistance to anoikis in human prostate cancer cell lines. Mol Cancer 6, 34 (2007).
- Kaini, R. R., Shen-Gunther, J., Cleland, J. M., Greene, W. A. & Wang, H.-C. Recombinant Xeno-Free Vitronectin Supports Self-Renewal and Pluripotency in Protein-Induced Pluripotent Stem Cells. Tissue Engineering Part C: Methods 22, 85–90 (2016).
- Rowland, T. J. et al. Roles of Integrins in Human Induced Pluripotent Stem Cell Growth on Matrigel and Vitronectin. Stem Cells and Development 19, 1231–1240 (2010).
- Sohi, A. N. et al. Synergistic effect of co-immobilized FGF-2 and vitronectin-derived peptide on feeder-free expansion of induced pluripotent stem cells. Materials Science and Engineering: C 93, 157–169 (2018).
- Ahn, S. et al. Engineering fibronectin-templated multi-component fibrillar extracellular matrices to modulate tissue-specific cell response. Biomaterials 308, 122560 (2024).
- Zhu, Z. et al. Zika Virus Targets Glioblastoma Stem Cells through a SOX2-Integrin αvβ5 Axis. Cell Stem Cell 26, 187-204.e10 (2020).
- Wijler, L. A. et al. Onward Spread from Liver Metastases Is a Major Cause of Multi-Organ Metastasis in a Mouse Model of Metastatic Colon Cancer. Cancers 16, 1073 (2024).
- Juhásová, J. et al. Osteogenic Differentiation of Miniature Pig Mesenchymal Stem Cells in 2D and 3D Environment. Physiol Res 559–571 (2011) doi:10.33549/physiolres.932028.
- Papo, N., Silverman, A. P., Lahti, J. L. & Cochran, J. R. Antagonistic VEGF variants engineered to simultaneously bind to and inhibit VEGFR2 and α v β 3 integrin. Proc. Natl. Acad. Sci. U.S.A. 108, 14067–14072 (2011).
- Richards, S., Leavesley, D., Topping, G. & Upton, Z. Development of Defined Media for the Serum-Free Expansion of Primary Keratinocytes and Human Embryonic Stem Cells. Tissue Engineering Part C: Methods 14, 221–232 (2008).
- Jensen, J. K., Wind, T. & Andreasen, P. A. The vitronectin binding area of plasminogen activator inhibitor‐1, mapped by mutagenesis and protection against an inactivating organochemical ligand. FEBS Letters 521, 91–94 (2002).