The motilin receptor is a heterotrimeric G-protein-coupled receptor (GPCR38) involved with regulating stomach motility.1,2 Since its discovery in 1999 researchers have been keen to develop compounds that can modify this receptor.3 In this article, we will explore everything that is known about its function, physiology, and pharmacology.
Stomach motility (motilin) model
This assay investigates whether your test article causes a change in contractile force in electrically stimulated stomach tissue.
Structure of the motilin receptor
The primary structure of the human motilin receptor consists of 412 amino acids.4,5 Its tertiary structure resembles a golf club consisting of seven transmembrane domains.4 The N-terminal is responsible for the binding capacity of the receptor, while the C-terminal protects the protein from enzymatic degradation.5
Location of the motilin receptor
The motilin receptor gene is located on human chromosome 13 q14-q21 and its RNA has been discovered in the duodenum, jejunum, and colon.4 The receptor protein is most densely expressed on smooth muscle cells within the stomach ("m" receptor subtype) and enteric nerves that are intrinsic to the stomach ("n" receptor subtype).4
Function of the motilin receptor
The primary function of the motilin receptor is to help contract smooth muscle cells in the stomach and small intestine during the migrating motor complex (MMC).4
What is the migrating motor complex (MMC)?
The MMC is a series of contractions that occur during fasting and are abolished by food intake.6 Input from the enteric nervous system is necessary to coordinate the MMC, alongside hormones that regulate and initiate this complex.6 The MMC contractions occur in three phases;7
- motor quiescence
- irregular contractions
- phasic propagating contractions
The motilin receptor is mainly involved in the final phase, where N-type receptors activate muscarinic cholinergic neurons in the distal antral pump of the stomach.7 The resultant contractions grind and sieve food into the small intestine, preventing bacterial overgrowth7 and preparing the gut for feeding.8
What is Motilin (MLN)?
Motilin (MLN) is a digestive hormone produced by enteroendocrine cells in the proximal small intestine.2,9,10 It is synthesized as an inactive prohormone consisting of a mature MLN molecule, signalling peptide, and C-terminal motilin-associated peptide (MAP).11 Secretion of MLN is cyclical9 increasing during fasting and then decreasing when phase III is abolished.12 While MLN's primary role is stimulating gut motility, it also triggers the secretion of pepsin and pancreatic enzymes.2,9,10 Additionally, MLN influences contractions of the gallbladder and lower esophageal sphincter (LES).2
Contraction in human Lower Esophageal Sphincter
This assay determines whether your test article affects the contraction of lower esophageal sphincter (LES) muscle strips.
We do not yet fully understand the full range of activity possessed by MLN1, or which intracellular cascades it triggers.13 We know that desensitization of the receptor occurs following phosphorylation (most likely by a GPCR kinase) which leads to receptor internalization and recycling.13 However, more research is needed to fully understand the intercellular signalling cascades associated with MLN.
Pharmacology of the motilin receptor
While no pathophysiological role has been linked to the motilin system, the motilin receptor and MLN expression are altered in some diseases.1 For example, decreased plasma levels of MLN are observed in patients with constipation and impaired stomach motility, while patients with irritable bowel syndrome (IBS) and gastroparesis diabeticorum (GD)14 show increased plasma levels of MLN.1,2
Motilin receptor agonists are currently used in acute care to prepare patients for rapid intubation or endoscopy1, however, they are not suitable for the treatment of chronic conditions.3 As there remains a clinical need for safe and effective gastric prokinetic agents suitable for long-term use1,15 pharmacologists are keen to develop novel motilin mimetics that can fulfil this unmet need.3
Erythromycin
Of all the motilin agonists used clinically, Erythromycin is the most researched in the literature. Erythromycin is a high-affinity analog of MLN10 that binds to motilin receptors on gastric and intestinal smooth muscle cells16 resulting in contraction.2 It belongs to the "motilide" drug class because it is both a macrolide antibiotic16 and an MLN agonist.2
Erythromycin is most commonly used as an antibiotic, however, it can also be used to reduce nausea or vomiting and to increase gastric motility.16 These prokinetic doses are typically lower,4 but it depends on the aim of the treatment.16 A low dose (0.5-1mg/kg) will increase peristalsis in the stomach and small intestine, which resembles phase III of the MMC.14 A higher dose (3mg/kg) will result in more rapid stomach emptying, but can also cause stomach cramps, diarrhea, and vomiting.16
Unfortunately, erythromycin is not suitable for the long-term treatment of gastroparesis16 as extended use of this drug risks antibiotic resistance and a decrease in prokinetic efficacy (i.e., tachyphylaxis).14 It is thought that the down-regulation of the motilin receptor is responsible for this observed tachyphylaxis in the long-term use of erythromycin.14
Stomach motility (Erythromycin) model
Investigate if your test article changes contractile force in stomach tissue, using erythromycin as a control compound.
Motilin Mimetics
Due to the issues surrounding the long-term use of erythromycin, researchers are keen to develop novel MLN mimetics17 which could be used to treat a range of conditions from GD1 to constipation-predominant IBS.15
Unfortunately, despite promising results in early studies, no novel MLN mimetics have successfully passed clinical trials.17 Both ABT-229 and SK-896 failed to display adequate efficacy in humans; the former because of tachyphylaxis and the latter due to species differences in canine models.17 The most clinical success was demonstrated by camicinal (GSK962040)9 which entered phase II clinical trials for the treatment of gastroparesis but then failed to progress any further in development.17
Models for investigating motilin receptor pharmacology
To develop new motilin receptor agonists, researchers require access to models that can predict efficacy at an early stage. Several nonclinical models have been developed for investigating the physiology and pharmacology of the motilin receptor, however, these vary in their translatability and there are advantages and disadvantages to each.
In vitro models
In vitro models are scalable, inexpensive systems that can be used to investigate the effect of new drugs on intracellular signalling cascades. Several of these models have been used to investigate novel motilin mimetics including cell-based tachyphylaxis, FLIPR (e.g., HeLa-MR9, CHO HEK293), and aequorin assays (CHO).1 However, researchers have noted variations in the kinetic response of motilin receptor agonists depending on the cell type used.1
In vivo models
Nonclinical in vivo models look at the effect of drugs in living, whole-body systems including small and large animals. Rodents (including rats, mice, and guinea pigs) do not have motilin receptors1,4 or a functional motilin system3 so are not used to study the effect of motilin receptor agonists.
Rabbits, on the other hand, have cerebral motilin receptors that closely resemble those found in humans.1,4 Studies in isolated rabbit gastric antrum have also been used to study motilin receptor pharmacology.1,4 However, the motilin receptors located in rabbit gastric antrum have a different structure and cellular location from the human motilin receptors, plus are rapidly desensitized ex vivo.1,4
Therefore, canine models are often the model of choice for studying motilin receptor activity. Yet the potency of motilin receptor agonists still varies between humans and dogs.3 There have even been cases where canine efficacy data does not translate clinically; for example, the motilin mimetic SK-896 caused increased GI motility in canine studies but did not show the same effect in humans.17 Species differences, such as variations in motilin receptor structure4 and MLN release2, are most likely responsible for these observed differences in pharmacology.
Overcoming species differences
So far, we have explored how the structure and function of MLN and the motilin receptor vary widely between non-human species4,8 as does receptor pharmacology.3 Functionally, MLN shows species differences in colonic motility, gastric emptying, and water/salt absorption in the small intestine.8 The effects of drugs that modify motilin receptor activity, such as erythromycin and TZP-201, are also species-dependent.1
Until more is understood, it is advisable to profile new motilin receptor drugs in isolated tissues that express the native receptor in a relevant cell type.1 This can be achieved preclinically in ex vivo studies that investigate motilin agonists in whole tissue.
Ex vivo models
Ex vivo models use living human tissue to isolate the response of an organ or tissue to a drug. To assess the effect of a compound on motilin receptor activity ex vivo, human stomach tissue is typically used - negating any species differences and generating human drug response data at an early stage.
One disadvantage of using stomach tissues in these studies is that the motilin receptors on nerves are not considered; however, the use of electric field stimulation (EFS) can solve this problem.1 EFS ensures that the motilin receptors are coupled to a functional nerve so that the tissues can more accurately assess the ability of novel drugs to maintain the facilitation of cholinergically-mediated contractions.1
Stomach motility (motilin) model
This assay investigates whether your test article causes a change in contractile force in electrical-field stimulated (EFS) stomach tissue.
Unfortunately, ex vivo research using human tissues can also be difficult to access. Not only is this research dependent on tissue supply, but there is donor consent and the anonymization of data to consider. There are CROs that specialize in ex vivo research, making it easier to access. If you would like to explore the effect of your test article on stomach tissue you can, therefore, outsource this work.
Conclusion
The motilin receptor plays an essential role in coordinating phase III contractions of the MMC during fasting. Little is known however about the intracellular signalling cascade triggered by its activation, and there are only a few motilin receptor agonists on the market that are only suitable for short-term use.
While several models exist for examining the activity of this receptor preclinically, animal models are limited by species differences and cell-based models show variation depending on the cell type used. Human tissue models, such as electrical field stimulation of strips of human stomach, provide more translational results, but are not easily accessible. CROs that specialize in this type of work can be used to provide easier access to translational drug discovery data.
At REPROCELL our human tissue experts can investigate the effect of your compounds on any gastrointestinal tissues, including esophageal, intestinal, and stomach tissues. To find out more information, please contact us via the inquiry form on our website.
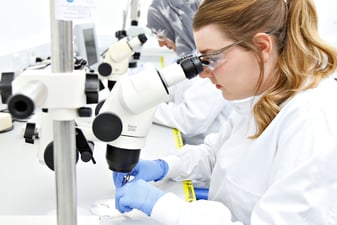
Preclinical and drug discovery CRO
Our contract research services use human living tissues to better predict drug safety and efficacy.
References
- Westaway SM et al. The Identification of and Rationale for Drugs Which Act at the Motilin Receptor. Progress in Medicinal Chemistry 48 pp 31-80 (2009).
- Englander EW et al. Postpyloric Gastrointestinal Peptides. Physiology of the Gastrointestinal Tract (2006).
- Leming S et al. GSK962040: a small molecule motilin receptor agonist which increases gastrointestinal motility in conscious dogs. Neurogastroenterology & Motility (2011).
- Poitras P. Motilin. Handbook of Biologically Active Peptides (2013).
- Kaiya H. Ghrelin-Motilin Family. Handbook of Hormones (2016).
- Olsson et al. The control of gut motility. Comparative Biochemistry and Physiology. 128 pp 481-503 (2001).
- Surjanhata et al. Gastrointestinal Motility and Enteric Neuroscience in Health and Disease. Reference Module in Biomedical Sciences (2014).
- Taheri S et al. Gastrointestinal Hormones and Tumor Syndromes. Endocrinology (2010).
- Shim J et al. Gut Hormones in Pregnancy and Lactation. Maternal-Fetal and Neonatal Endocrinology (2020).
- Vella A et al. Gastrointestinal Hormones and Gut Endocrine Tumours. Williams Textbook of Endocrinology (2011).
- Sakai T et al. Motilin. Physiology of the Gastrointestinal Tract (2006).
- Rickesha LW et al. Anatomy and Physiology of the Stomach. Shakelford's Surgery of the Alimentary Tract (2019).
- Sakai T. Motilin. Handbook of Hormones (2016).
- Jain R et al. Gastrointestinal and Hepatic Manifestations of Systemic Diseases. Sleisenger and Fordtran's Gastointestinal and Liver Disease (2010).
- Vella A et al. Gastrointestinal Hormones and Neurotransmitters. Sleisenger and Fordtran's Gastointestinal and Liver Disease (2010).
- Feldman M. Nausea and Vomiting. Sleisenger and Fordtran's Gastrointestinal and Liver Disease (2021).
- Lafferty RA et al. Pharmacology of Gut Hormone Mimetics for Obesity and Diabetes. Reference Module in Biomedical Sciences (2021).